what criteria would you use to determine if a protein was exhibiting prion properties?
Abstract
Prion-like behaviour is attracting much attending due to the growing evidences that amyloid-like self-assembly may reach across neurodegeneration and exist a conserved functional machinery. The best characterized functional prions stand for to a subset of yeast proteins involved in translation or transcription. Their conformational promiscuity is encoded in Prion Forming Domains (PFDs), unremarkably long and intrinsically matted poly peptide segments of depression complexity. The compositional bias of these regions seems to exist important for the transition between soluble and amyloid-like states. We have proposed that the presence of cryptic soft amyloid cores embedded in yeast PFDs can likewise be important for their associates and demonstrated their being and self-propagating abilities. Here, we used an orthogonal approach in the search of man domains that share yeast PFDs compositional bias and exhibit a predicted nucleating cadre, identifying 535 prion-similar candidates. We selected seven proteins involved in transcriptional or translational regulation and associated to disease to characterize the backdrop of their amyloid cores. All of them self-assemble spontaneously into amyloid-like structures able to propagate their polymeric country. This provides back up for the presence of short sequences able to trigger conformational conversion in prion-like human proteins, potentially regulating their functionality.
Introduction
A broad range of human pathologies, ranging from neurodegenerative conditions such equally Alzheimer's and Parkinson's diseases, to non-neuronal disorders such equally type 2 diabetes and cataracts, are associated with protein misfolding and aggregation into amyloid-like structures1. The self-assembly of proteins into β-sheet-enriched amyloid conformations appears to obey, in many cases, the and then-called amyloid-short-stretch hypothesis, according to which, assemblage is commencement nucleated past the intermolecular contacts formed past a reduced number of specific short regions in the protein2. In pathogenic proteins, these stretches are generally effectually 5–10 residues in length, with a high assemblage propensity and a predominant hydrophobic characterthree.
Prions are proteins able to adopt multiple structural conformations, from which at least one has self-propagating properties, usually an amyloid state4. Prions have been traditionally associated with the onset of mammalian neurophatologies5. Nevertheless, there are evidences that prion-like mechanisms are not always deleterious and instead they can exist used for benign purposessix. The best characterized gear up of functional prions has been institute in yeast, where they can behave as epigenetic elements, facilitating adaptation to fluctuating environments7,eight. The conformational promiscuity of yeast prions is encoded in Prion Forming Domains (PFDs)9. PFDs are both sufficient and necessary for prion conversion and usually stand for to long and intrinsically disordered segments of low complexity9.
The information on the common features shared by yeast PFDs has fueled the development of algorithms aimed to identify like prion-like domains (PrLDs) and the proteins that contain them at the proteome level9,10,11,12,13,14,xv,16,17. Despite PFDs bear the chapters to shift to an amyloid country, classical amyloid prediction algorithms fail to identify them12. This observation led to suggest that PFDs assembly is governed past the low complication and compositional bias common to these domains9,12. Examples of algorithms exploiting these features are DIANAten, LPS11, PrionScan13, PLAAC16 and PAPA12. Remarkably, these computational approaches predict the being of PrLD-containing proteins in a wide variety of organisms, from prokaryotes to college eukaryotes18,19,20,21,22,23. In humans, this sub-proteome is enriched in nucleic acid-binding proteins18,19. A fraction of these proteins seem to be involved in the formation of membraneless intracellular compartments, similar RNA granules, through PrLDs mediated liquid-liquid phase separation24,25. Mutations in these domains have been shown to promote an aberrant transition to an aggregated amyloid-like state, the formation of which might led to the onset of neurodegenerative diseases26,27.
We take recently proposed that, in improver to limerick, the presence of soft amyloid cores within PFDs and PrLDs could exist important for their associates28,29. We rationalized that these assembly-nucleating regions should be longer than classical amyloid stretches, in such a way that the amyloid potential would exist more diffusely distributed; each residue having an boilerplate lower potency, but with more residues contributing to the assembling force. This will make their aggregation sensitive to protein concentration and seeding. Thus, in our view, the assemblage of prion-similar domains shares mechanistic features with those of classical amyloidogenic proteins. This notion was implemented in pWALTZ, an algorithm that predicts the 21-residues long sequence stretch with the highest average amyloid potential in PrLDs14,15. This concept was farther validated experimentally past demonstrating the existence of such soft amyloid stretches in the prion domains of four of the best characterized yeast prions30, as well equally in the predicted PrLD of the Rho termination factor of Clostridium botulinum 31, which later on led to the discovery of the first bacterial prion-similar protein32.
Here, we written report the presence of soft amyloid cores in novel putative human being prion-similar proteins. For this purpose, we performed a stringent computational assay of the human proteome in the search of domains that, while fulfilling the compositional bias characteristic of PrLDs, would also exhibit a sequence stretch that tin can potentially nucleate their self-assembly. From this set up, nosotros selected seven nucleic acid-binding proteins associated to illness (DDX5, EYA1, ILF3, MED15, NCOA2, PHC1 and TIA1) to structurally characterize the nature of their putative nucleating cores. The results herein indicate that the PrLDs of all these proteins include 21-residues long stretches able to cocky-get together spontaneously into non-toxic, β-canvass enriched, Thioflavin-T positive amyloid-like structures displaying self-seeding activity. Therefore, the nowadays work provides compelling experimental show for the beingness of specific sequences with the potential to trigger the conformational conversion of PrLDs in human proteins.
Results
Identification of PrLD soft amyloid cores in human prion-similar proteins
The analysis of the 70940 protein sequences in the human proteome was initially performed with PAPA12 and further refined with pWALTZfourteen. Both PAPA and pWALTZ algorithms were trained on summit of yeast prions; nonetheless, they are based on radically different concepts, a suitable composition of the PrLD and the presence of an embedded soft amyloid core, respectively. Sequences identified by these 2 orthogonal approaches are expected to recapitulate the conformational backdrop of yeast prions. A total of 663 human proteins were identified past PAPA and afterwards shortlisted using pWALTZ to return a total of 535 polypeptides, encoded past 336 different genes (Table S1).
We wanted to test whether the soft amyloid cores predicted inside the PrLDs of these putative man prion-like proteins could spontaneously self-gather into amyloid-like conformations and propagate their aggregated state, every bit we observed before for yeast prions30 and the prion-similar C. botulinum Rho gene31. We focused on nucleic acid-binding proteins, both considering this molecular office is enriched in our dataset and because near of the experimentally validated yeast prions human activity in translational or transcriptional regulation. We selected six proteins associated to disease whose prionogenic properties have non been reported before: DDX5, EYA1, ILF3, MED15, NCOA2 and PHC1. Nosotros also included in the analysis TIA1, an RNA-bounden poly peptide identified by the orthogonal arroyo for which a prion-like behavior has been already suggested33 (Tables ane and 2).
DDX5 (p68) (Fig. 1A) is a member of the Expressionless box family of RNA helicases involved in transcriptional regulation34 and it is overexpressed in various types of cancers such as those of prostate, chest and colon; promoting prison cell proliferation and metastasis35,36. The C-terminal region containing the predicted PrLD seems to play a office in the interaction of the protein with other components of the transcriptional machinery37.
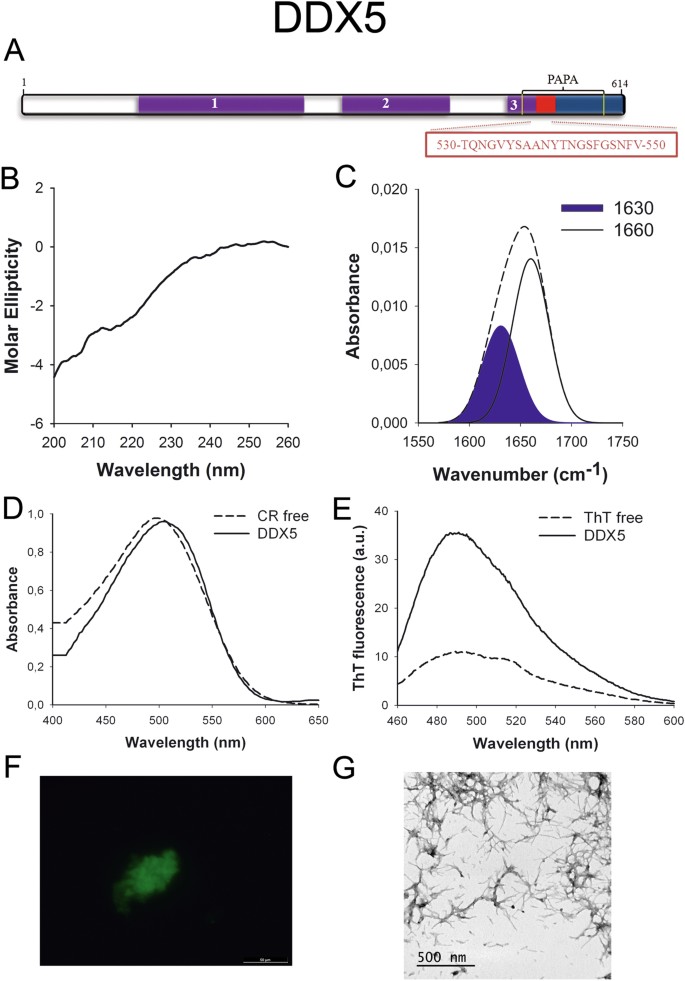
DDX5 PrLD amyloid cadre. (A) DDX5 diagram showing the location of the identified Pfam domains (majestic), the amyloid cadre (red) and the PrLD equally predicted by PLAAC (blue) and PAPA (xanthous lines). 1 = DEAD domain. two = Helicase C domain. 3 = p68-similar RNA helicase domain. The sequence of the amyloid cadre is shown in the box. (B) CD spectrum in the far-UV region of 100 μM DDX5 peptide in 5 mM potassium phosphate buffer pH 7.4 before incubation. (C) DDX5 peptide FT-IR absorbance spectrum in the amide I region. The dashed line corresponds to the original spectrum, the blue area indicates the contribution of the inter-molecular β-canvas signal to the total area upon Gaussian deconvolution. (D) CR absorbance spectrum in the absenteeism (dashed line) and in the presence (solid line) of DDX5 peptide. (East) Fluorescence emission spectrum of Th-T in the absence (dashed line) and in the presence (solid line) of DDX5 peptide. (F) DDX5 peptide stained with Th-S and observed at 40X magnification using fluorescence microscopy. (G) DDX5 peptide representative manual electron micrograph. The data in panels C to G were nerveless upon incubation of DDX5 peptide for 2 days in 5 mM potassium phosphate buffer pH vii.iv at 37 °C.
EYA1 (Fig. 2A) is a transcriptional coactivator and a poly peptide phosphatase with regulatory roles in nephrogenesis38. The protein is overexpressed in breast cancer39 and mutations in EYA1 factor are associated with branchio-oto-renal syndromeforty. The North-terminal region containing the PrLD has been reported to office equally a transactivation domain41.
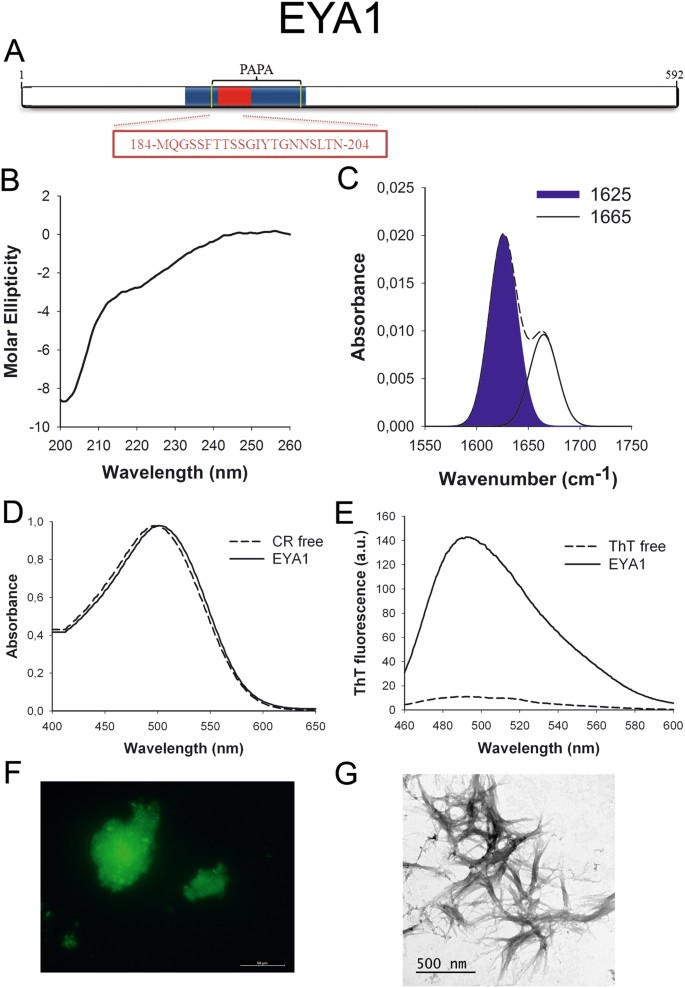
EYA1 PrLD amyloid core. (A) EYA1 diagram showing the location of the amyloid core (red) and the PrLD as predicted by PLAAC (blue) and PAPA (yellow lines). The sequence of the amyloid cadre is shown in the box. (B) CD spectrum in the far-UV region of 100 μM EYA1 peptide in 5 mM potassium phosphate buffer pH vii.4 before incubation. (C) EYA1 peptide FT-IR absorbance spectrum in the amide I region. The dashed line corresponds to the original spectrum, the blueish expanse indicates the contribution of the inter-molecular β-sail betoken to the full area upon Gaussian deconvolution. (D) CR absorbance spectrum in the absence (dashed line) and in the presence (solid line) of EYA1 peptide. (E) Fluorescence emission spectrum of Th-T in the absence (dashed line) and in the presence (solid line) of EYA1 peptide. (F) EYA1 peptide stained with Thursday-South and observed at 40X magnification using fluorescence microscopy. (Thousand) EYA1 peptide representative transmission electron micrograph. The data in panels C to G were collected upon incubation of EYA1 peptide for ii days in 5 mM potassium phosphate buffer pH vii.4 at 37 °C.
ILF3 (Fig. 3A) works in RNA metabolism, from transcription to deposition and it appears to be essential for cellular development42. Interestingly, ILF3 participates in ribonucleoprotein (RNP) granules assembly43 and it interacts with FUS, a well-characterized prion-like protein44.
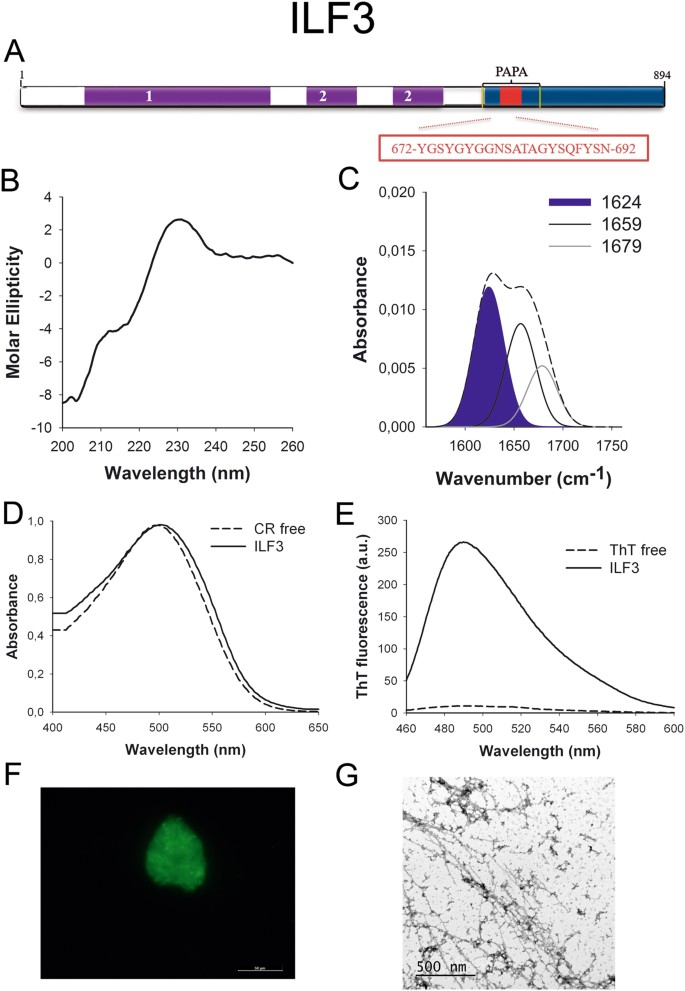
ILF3 PrLD amyloid cadre. (A) ILF3 diagram showing the location of the identified Pfam domains (majestic), the amyloid cadre (blood-red) and the PrLD as predicted by PLAAC (blue) and PAPA (yellow lines). 1 = DZF domain. 2 = dsRNA binding motif. The sequence of the amyloid core is shown in the box. (B) CD spectrum in the far-UV region of 100 μM ILF3 peptide in 5 mM potassium phosphate buffer pH 7.four before incubation. (C) ILF3 peptide FT-IR absorbance spectrum in the amide I region. The dashed line corresponds to the original spectrum, the blue area indicates the contribution of the inter-molecular β-sheet betoken to the total area upon Gaussian deconvolution. (D) CR absorbance spectrum in the absence (dashed line) and in the presence (solid line) of ILF3 peptide. (East) Fluorescence emission spectrum of Th-T in the absence (dashed line) and in the presence (solid line) of ILF3 peptide. (F) ILF3 peptide stained with Th-S and observed at 40X magnification using fluorescence microscopy. (Thou) ILF3 peptide representative transmission electron micrograph. The data in panels C to G were collected upon incubation of ILF3 peptide for 2 days in 5 mM potassium phosphate buffer pH 7.four at 37 °C.
MED15 (Fig. 4A) is ane office of the Mediator complex involved in the transcription of RNA-polymerase II dependent genes45. The identified PrLD corresponds to a Q-rich region similar to those accounting for conformational conversion in yeast prions. Indeed, its yeast homolog has been already classified as a prion46 with the ability to form amyloid-like structures in vivo under stress weather47.
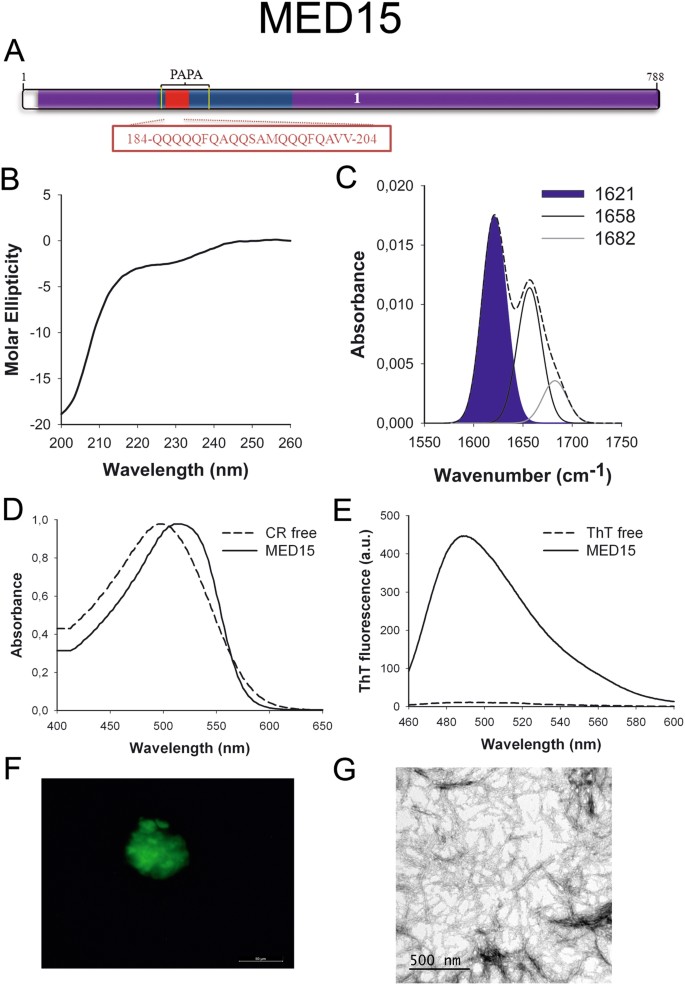
MED15 PrLD amyloid core. (A) MED15 diagram showing the location of the identified Pfam domains (royal), the amyloid core (cherry) and the PrLD equally predicted past PLAAC (blue) and PAPA (yellowish lines). 1 = MED15 domain. The sequence of the amyloid core is shown in the box. (B) CD spectrum in the far-UV region of 100 μM MED15 peptide in five mM potassium phosphate buffer pH seven.4 before incubation. (C) MED15 peptide FT-IR absorbance spectrum in the amide I region. The dashed line corresponds to the original spectrum, the blue area indicates the contribution of the inter-molecular β-canvas signal to the total area upon Gaussian deconvolution. (D) CR absorbance spectrum in the absence (dashed line) and in the presence (solid line) of MED15 peptide. (East) Fluorescence emission spectrum of Th-T in the absenteeism (dashed line) and in the presence (solid line) of MED15 peptide. (F) MED15 peptide stained with Th-S and observed at 40X magnification using fluorescence microscopy. (G) MED15 peptide representative transmission electron micrograph. The data in panels C to Yard were collected upon incubation of MED15 peptide for 2 days in v mM potassium phosphate buffer pH 7.4 at 37 °C.
NCOA2 (Fig. 5A) is a transcriptional coactivator for steroid receptors and nuclear receptors acting in the upregulation of Dna expression48. It has a tissue-specific role in tumorigenesis, acting as an oncogene in prostate cancer49 and as a tumor suppressor in liver cancer50. Moreover, NCOA2 is a fundamental player in glucose homeostasis, being involved in Mediator recruitment for glucokinase expression51.
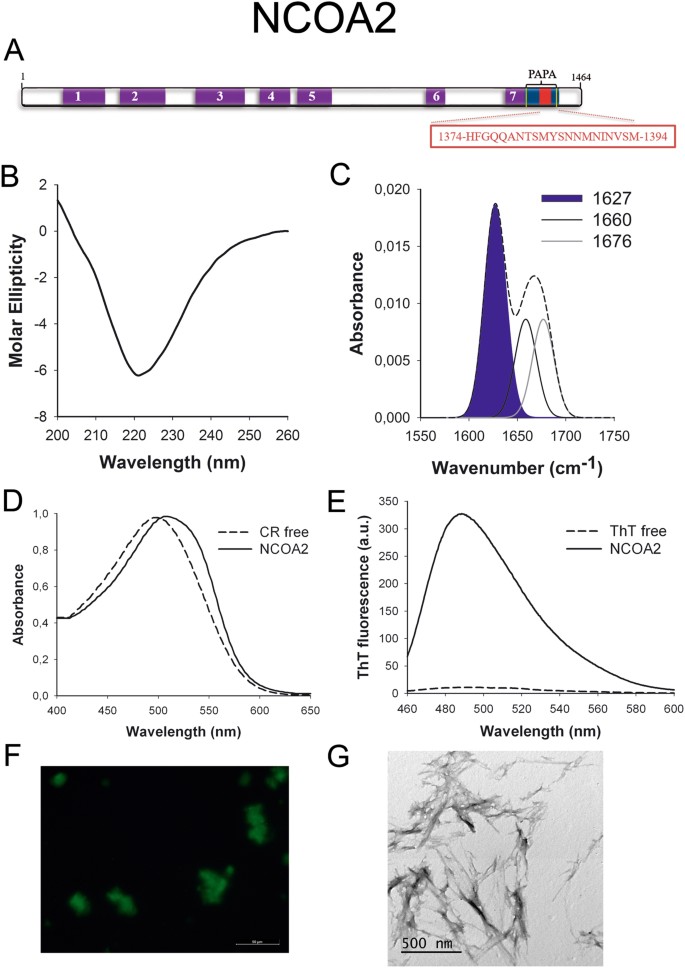
NCOA2 PrLD amyloid core. (A) NCOA2 diagram showing the location of the identified Pfam domains (purple), the amyloid core (carmine) and the PrLD every bit predicted by PLAAC (blue) and PAPA (yellow lines). 1 = PAS domain. ii = PAS11 domain. 3 = NCOA_u2 domain. 4 = SRC1 domain. five = Duf4927 domain. six = Nuc rec co-act domain. 7 = DUF1518. The sequence of the amyloid core is shown in the box. (B) CD spectrum in the far-UV region of 100 μM NCOA2 peptide in 5 mM potassium phosphate buffer pH 7.4 earlier incubation. (C) NCOA2 peptide FT-IR absorbance spectrum in the amide I region. The dashed line corresponds to the original spectrum, the blue area indicates the contribution of the inter-molecular β-sheet signal to the full expanse upon Gaussian deconvolution. (D) CR absorbance spectrum in the absence (dashed line) and in the presence (solid line) of NCOA2 peptide. (E) Fluorescence emission spectrum of Th-T in the absence (dashed line) and in the presence (solid line) of NCOA2 peptide. (F) NCOA2 peptide stained with Th-S and observed at 40X magnification using fluorescence microscopy. (One thousand) NCOA2 peptide representative transmission electron micrograph. The data in panels C to G were collected upon incubation of NCOA2 peptide for 2 days in 5 mM potassium phosphate buffer pH 7.iv at 37 °C.
PHC1 (Fig. 6A) is ane component of the Polycomb complex responsible for cellular differentiation during evolution. This complex is constituted past factor silencing proteins that repress important developmental regulator genes, including homeotic (HOX) genes52. PHC1 is associated with primary microcephaly53.
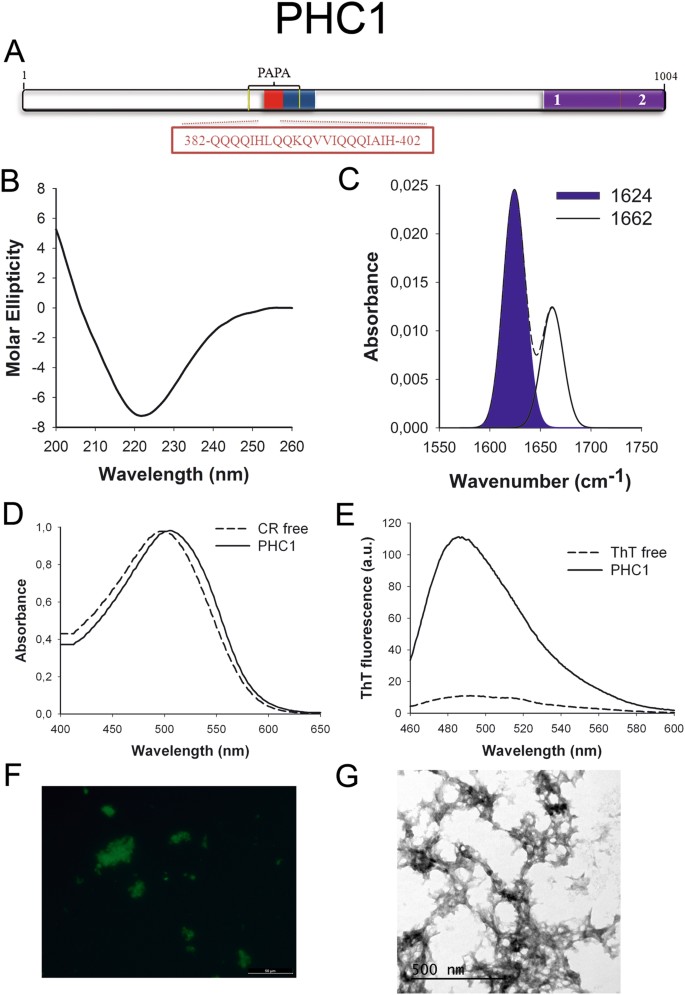
PHC1 PrLD amyloid core. (A) PHC1 diagram showing the location of the identified Pfam domains (imperial), the amyloid cadre (red) and the PrLD as predicted past PLAAC (bluish) and PAPA (yellow lines). 1 = PHC2 SAM assoc domain. ii = SAM1 domain. The sequence of the amyloid core is shown in the box. (B) CD spectrum in the far-UV region of 100 μM PHC1 peptide in v mM potassium phosphate buffer pH vii.4 before incubation. (C) PHC1 peptide FT-IR absorbance spectrum in the amide I region. The dashed line corresponds to the original spectrum, the blue area indicates the contribution of the inter-molecular β-canvas indicate to the total area upon Gaussian deconvolution. (D) CR absorbance spectrum in the absence (dashed line) and in the presence (solid line) of PHC1 peptide. (Due east) Fluorescence emission spectrum of Thursday-T in the absence (dashed line) and in the presence (solid line) of PHC1 peptide. (F) PHC1 peptide stained with Th-Due south and observed at 40X magnification using fluorescence microscopy. (G) PHC1 peptide representative transmission electron micrograph. The information in panels C to Yard were collected upon incubation of PHC1 peptide for two days in five mM potassium phosphate buffer pH vii.4 at 37 °C.
TIA1 (Fig. 7A) is an RNA-binding protein and a component of stress granules required to regulate culling splicing and mRNA translation and turnover. The predicted PrLD lies in a Q-rich region. TIA1 has already been suggested to be a functional prion-similar protein whose dysregulation is involved in Amyotrophic Lateral Sclerosis (ALS)33. All the same, the presence of an amyloid core inside its PrLD has not been addressed before.
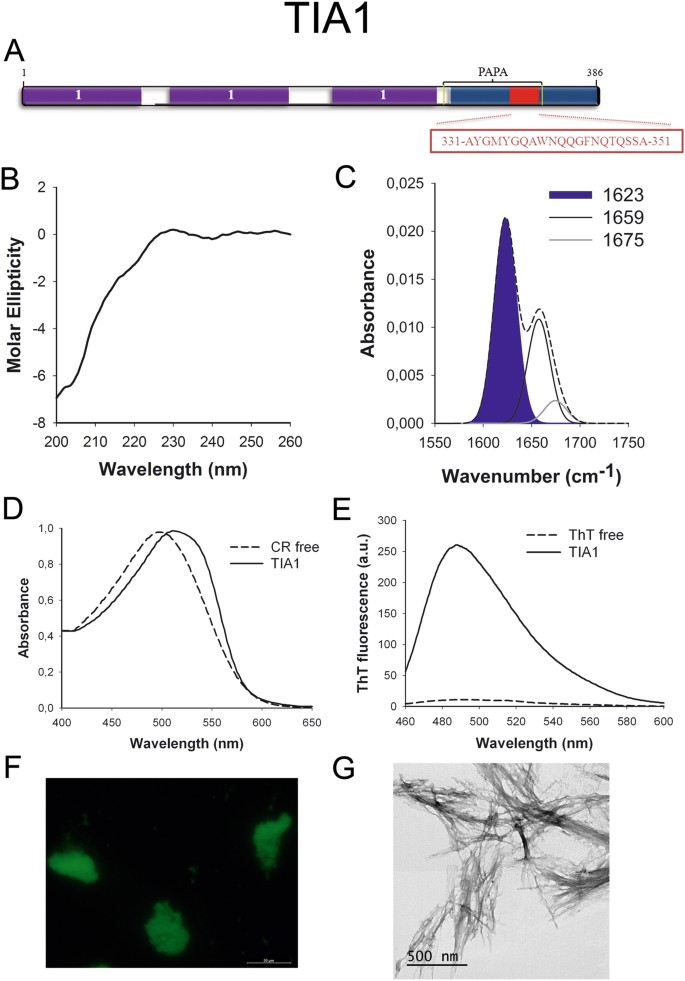
TIA1 PrLD amyloid core. (A) TIA1 diagram showing the location of the identified Pfam domains (purple), the amyloid cadre (red) and the PrLD as predicted past PLAAC (blue) and PAPA (yellow lines). i = RNA recognition motif (RRM). The sequence of the amyloid core is shown in the box. (B) CD spectrum in the far-UV region of 100 μM TIA1 peptide in 5 mM potassium phosphate buffer pH 7.four before incubation. (C) TIA1 peptide FT-IR absorbance spectrum in the amide I region. The dashed line corresponds to the original spectrum, the bluish expanse indicates the contribution of the inter-molecular β-sheet signal to the full area upon Gaussian deconvolution. (D) CR absorbance spectrum in the absence (dashed line) and in the presence (solid line) of TIA1 peptide. (East) Fluorescence emission spectrum of Th-T in the absence (dashed line) and in the presence (solid line) of TIA1 peptide. (F) TIA1 peptide stained with Th-Southward and observed at 40X magnification using fluorescence microscopy. (One thousand) TIA1 peptide representative transmission electron micrograph. The information in panels C to M were collected upon incubation of TIA1 peptide for two days in 5 mM potassium phosphate buffer pH vii.4 at 37 °C.
To further confirm the presence of PrLDs in these proteins and to define more precisely their boundaries we used PLAAC, all the same another composition-based predictor, in which, in contrast to PAPA, the length of the predicted PrLD also depends on the poly peptide composition. PLAAC detected PrLDs, overlapping with the regions previously identified by PAPA, in all the higher up described proteins (Figs 1A to 7A).
The predicted soft amyloid cores for these proteins are shown in Table 2. Remarkably, well-validated aggregation predictors like Aggrescan54, Tango55 and Zyggregator56 failed to classify these stretches as aggregation-prone, the exception being the PHC1 core, which was identified by Tango (Table S2). The underlying reason explaining why these amyloid predictors neglect to score properly the putative cores is likely their much lower hydrophaticity, when compared with the amyloid stretches present in pathogenic proteins similar Aβ42 or α-synuclein (Table S3). Indeed, the predicted cores are enriched in polar residues like Gln, Asn and Ser equally well equally in Gly (Table 2), all amino acids considered conferring low aggregation propensities to poly peptide sequences.
An assay of the structural context in which these soft amyloid cores are embedded in their respective sequences using the prediction algorithms FoldIndex57, IUPRED58, PondR-FIT59 and RONN60 indicates that they are preferentially located in matted protein segments (Table S4).
Predicted human PrLD soft amyloid cores get together into β-sheet rich structures
We synthetized 21-residues long peptides correspondent to the detected amyloid cores and analyzed their secondary construction content by far-UV circular dichroism (CD) immediately subsequently their dilution at 100 μM in sodium phosphate buffer at pH seven.4 (Figs 1B to 7B). Five out of the seven peptides (DDX5, EYA1, ILF3, MED15 and TIA1) exhibited spectra consequent with a mostly disordered conformation. In ILF3, the high content in Tyr residues (23%) renders a characteristic aromatic indicate at 230 nm. The other two peptides (NCOA2 and PHC1) already exhibited a β-canvas CD spectrum immediately after dilution, thus suggesting that they experience a very fast assembly in aqueous buffer.
Next, we incubated the peptides at 100 μM for 2 days at 37 °C and monitored their ability to course macromolecular structures using synchronous light scattering, bis-ANS bounden, infrared spectroscopy (ATR-FTIR) and far-UV CD.
The germination of loftier-order assemblies later incubation was confirmed for all peptides by measuring the lite handful of the correspondent solutions. All of them exhibited significant scattering indicate after ii days (Effigy S1). Despite the amyloid cores in this study are less hydrophobic than those of pathogenic amyloids (Tabular array S3), they yet showroom non-polar residues that might contribute to the initial assembly. We explored the presence of exposed hydrophobic clusters in the detected aggregated fabric by measuring their binding to bis-ANS (Figure S2A), a dye that increases its fluorescence emission upon interaction with these regions61. The bis-ANS fluorescence emission maximum increases and blueshifts from 530 nm in the absence of peptides to 510 nm in their presence, indicating the existence of hydrophobic-patches in the surface of all these assemblies. The binding of these assemblies to bis-ANS was, however, much lower than those of the amyloid fibrils formed past the Parkinson'due south associated α-synuclein poly peptide at the aforementioned concentration (Figure S2B), consistent with the lower hydropathicity of PrLDs amyloid stretches.
Next, we recorded the amide I region of the FTIR spectrum (1700–1600 cm−ane) for these aggregates (Figs 1C to 7C). This region corresponds to the absorption of the carbonyl peptide bond grouping of the protein main chain and it is conformation sensitive. Deconvolution of the spectra allowed us to assign the private secondary structure elements of incubated peptides and their relative contribution to the main absorbance (Table S5). In all the cases nosotros could identify a strong ring at 1620–1630 cm−1, usually assigned to the presence of inter-molecular β-sheets. This signal is the largest contributor to the absorbance spectrum in all peptides, except for DDX5, where it contributes 37% of the expanse. Interestingly, no anti-parallel β–canvas ring was detected (~1690 cm−i) in any of the samples; thus suggesting that the detected β–strands in cocky-assembled peptides would adopt preferentially a parallel disposition. The other detected structural elements are associated with disordered structure and turns (Table S5). We also monitored the secondary construction of the incubated peptides using far-UV CD (Figure S3). In all cases we could find a band at 215–220 nm consequent with the population of a β-sheet enriched conformation, despite in some cases the ellipticity was depression, indicating that a meaning proportion of the peptide was aggregated, therefore out of the solution and non detectable.
Overall, our data are consequent with the spontaneous associates of the predicted human PrLD amyloid cores into supramolecular β-sheet enriched structures.
Predicted human PrLD amyloid cores class not-toxic amyloid-similar fibrillar structures
We used the amyloid-specific dyes Congo cerise (CR), Thioflavin T (Th-T) and Thioflavin-Due south (Th-Due south) to confirm that the detected β–sheet enriched aggregates were organized into amyloid-similar suprastructures.
The absorbance spectra of CR red shifts in the presence of amyloid aggregates62. Incubation of CR in the presence of aggregated peptides resulted in a reddish shift of its spectrum in all the cases (Figs 1D to 7D); despite for DDX5, EYA1 and ILF3 the spectral shift was small. To confirm the ability of these three peptides to bind CR they were incubated under the same atmospheric condition at 500 μM final concentration. The peptides in these three solutions promoted a clear shift of the absorption maximum of the dice (Effigy S4).
Th-T fluorescence emission is enhanced in the presence of amyloid fibrils63. All the peptides promoted an increment in the intensity of Th-T fluorescence spectral maximum at 488 nm (Figs 1E to 7E). Furthermore, binding of Thursday-Due south to the aggregates could be visualized by fluorescence microscopy for all incubated peptides. Areas rich in fibrous textile were stained with Thursday-Southward to yield green-yellow fluorescence against a nighttime background in all cases (Figs 1F to 7F).
The dye binding results signal that incubated peptide solutions might contain detectable amounts of amyloid-similar structures. To confirm this extent, the morphological features of the peptide assemblies in these samples were analyzed using manual electron microscopy (TEM). Negative staining indicated that all peptides finer assemble into supramolecular structures (Figs 1G to 7G). The aggregates formed by DDX5, EYA1, MED15, NCOA2, and TIA1 stand for to amyloid-similar fibrillar arrangements, without any significant accumulation of amorphous cloth. The fibrils exhibit a diameter that varies from 5 to x nm and a length that ranges from 2 to 10 μm. In the ILF3 sample long amyloid-like fibrils coexist with minor aggregates that announced to attach to the fibrils, whereas, for PHC1, despite its amyloid-like tintorial properties, the material appears to be essentially protofibrillar. All the peptides were also able to form macromolecular aggregates when they were incubated at 10 μM (one/10 the concentration used in the previous assays) (Effigy S5).
The higher up data indicate that the predicted amyloid cores exhibit a strong propensity to form amyloid-like assemblies. The amyloids formed by pathogenic protein fragments are usually highly cytotoxic. We tested if the aggregates formed by the PrLD amyloid cores display any toxicity when administered to neuroblastoma SH-SY5Y cells. All the aggregates were essentially innocuous when added to the cell cultures at up to ten μM (Figures S6).
Predicted homo PrLD amyloid cores grade aggregates with self-seeding properties
Seeded protein aggregation is a well-established mechanism for in vivo amyloid fibril germination and underlies prion propagation64. The nucleation step of the amyloid associates is shortened in the presence of preformed amyloid fibrils of the same protein, that tin act as nuclei for the subsequent polymerization reaction65. Specific and short assemblage-prone regions have been shown to play a crucial function in this process66,67. To test whether preformed PrLDs core amyloid-similar assemblies can seed the aggregation of the correspondent soluble peptides, nosotros followed the aggregation kinetics of the peptides at 100 µM in the presence and absence of 2% (west/west) of preformed aggregates (Fig. 8). We could not monitor the assemblage kinetics of NCOA2 or PHC1 because they exhibited very high Thursday-T signal from the very beginning of the reaction, consequent with a very fast assembly into β-sheet structures, as suggested by the far-UV CD spectra they exhibit immediately upon dilution in aqueous solution (Figs 5B and 6B). The balance of peptides exhibited characteristic sigmoidal aggregation kinetics with lag-phases ranging from twenty to 120 min in the absenteeism of seeds. The add-on of preformed aggregates strongly accelerated the formation of Th-T positive assemblies in all cases (Fig. 8), supporting a nuclei-dependent aggregation machinery and raising the possibility that such specific interactions could too occur in the context of the complete proteins in which these short regions are embedded.
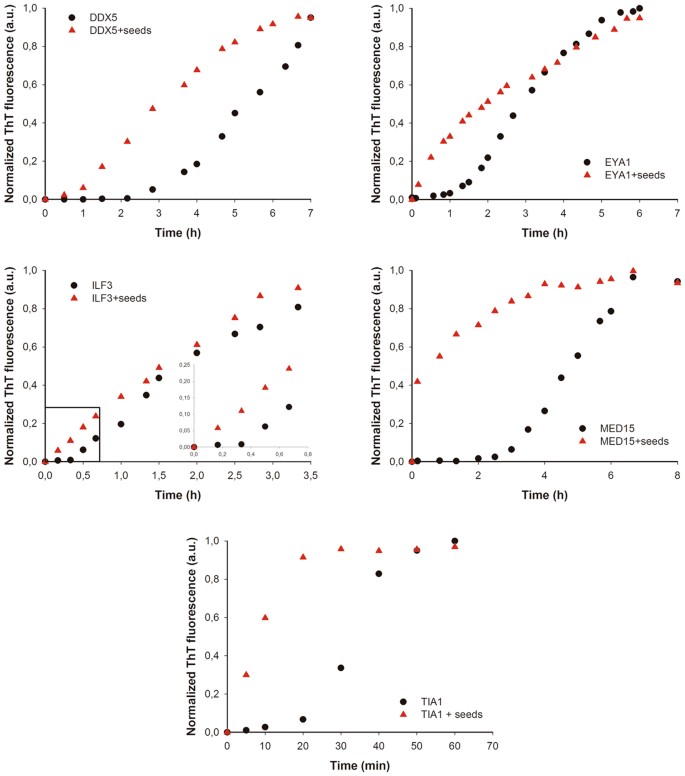
Human being PrLD amyloid cores kinetics. Aggregation kinetics of DDX5, EYA1, ILF3, MED15 and TIA1 peptides at 100 µM in the absence (black circles) and presence (cerise triangles) of 2% (w/w) of preformed aggregates (seeds) were monitored by tracking the changes in Th-T fluorescence emission spectra. All the peptides exhibited accelerated kinetics in the presence of seeds.
Discussion
The prion miracle is all-time known past its association with spongiform encephalopaties in mammals, but in the last years a growing list of non-pathological prion-like proteins are being discovered. Functional prions were originally identified in yeast68. In these prion proteins, specific PFDs encode for their ability to switch between soluble and cocky-assembled states. Computational analysis are revealing the existence of polypeptides displaying like domains in previously unexplored proteomes17. This suggests that this type of conformational conversion might exist an evolutionary conserved mechanism exploited past different organisms, including humans, for benign purposes.
A characteristic feature of many pathogenic amyloids is the presence of short hydrophobic sequence stretches able to nucleate the assembly of these proteins into toxic aggregates both in vitro and in vivo under pathological weather condition. These highly amyloidogenic regions seem to be absent-minded in PFDs and PrLDs, likely because their potency will unbalance the equilibrium betwixt the soluble and self-assembled states of the proteins towards an irreversibly aggregated and potentially toxic state, even in the absence of stress. Even so, nosotros accept recently proposed that in addition to a special compositional bias, PFDs and PrLDs contain indeed cryptic soft amyloid cores that can play an important function at the early stages of assembly past restricting inter-molecular interactions to specific regions of these long domains28. In these cores, the amyloid nucleating potency would be weaker and less concentrated that in pathogenic amyloids. This property would let the protein to remain soluble in most physiological atmospheric condition, while beingness responsive to conditions that favor inter-molecular contacts or to the presence of preformed assemblies that target these specific segments. We have provided evidences for the presence of such regions in the PFDs of four of the best characterized yeast prions and for the ability of 1 of these soft amyloid cores to recruit the assembly of its correspondent total-length PFD in vitro and in the cell30.
Using an arroyo analogous to the ane described here, we previously screened the C. Botulinum proteome for the presence of regions displaying both compositional similitude to bona fide prions and containing a soft amyloid cadre, identifying a first bacterial PrLD in the Rho Terminator factor of this pathogen31. After on, Yuan and Hochschild validated the prion-similar nature of this protein, demonstrating that Rho can access culling protein conformations in prokaryotes, including a self-assembled state with decreased Rho activity that results in genome-wide changes at the transcriptome level32. The seven human proteins selected in the nowadays study for experimental characterization, exhibit predicted PrLDs with the aforementioned compositional properties than Rho or the yeast prions and are too regulatory nucleic acid-binding proteins. We show here that they all incorporate a region able to apart self-assemble into amyloid-similar structures displaying low cytotoxicity, a property that is probable linked to the weak hydrophobic patches in these assemblies, compared with those present in the fibrils of pathogenic proteins like α-synuclein1. In addition, for all the cases we could test, the fibrillar assemblies had the capacity to seed and accelerate the aggregation reaction of their soluble counterparts. Despite our assays are in vitro and merely with a slice of the protein, the results reveal the self-assembly potential encoded in homo prion-similar proteins.
Like to Curt Linear Interaction Motifs (SLIMs) in intrinsically disordered proteins, the assembling properties of soft amyloid cores might contribute to mediate PrLDs functional protein-protein interactions (PPI)28. Actually, it is known that the interaction betwixt PrLDs of RNA granules proteins like TDP43, TAF15 and FUS is sufficient to induce liquid-liquid phase separation7, just likewise reversible hydrogels69, which take a high β-sheet content, but are labile to dilution. Interestingly plenty, mutations that severely impede the germination of intermolecular β-sheets also inhibit the ability of PrLDs to actuate transcription, indicating that, in this particular context, the germination of soft amyloid-like assemblies plays a functional role70. DDX5, ILF3 and TIA1 are also RNA granule proteins43,71,72,73; thus it is tempting to advise that the identified PrLDs and embedded soft amyloid cores might likewise play a role in the germination of functional β-canvass-containing assemblies. The other 4 proteins in our written report are either structural components of macromolecular complexes (MED15 and PHC1) or they are involved in poly peptide-poly peptide interactions (NCOA2 and EYA1). The detected PrLDs and the gluey nature of their cores might facilitate these contacts. It has been suggested that the role of PrLDs would be to promote a primary level of organisation through homotypic interactions and that, once oligomerized, these assemblies would facilitate the establishment of novel lateral interactions with other proteins74. Indeed, an analysis of the interaction networks of the vii proteins in this study using the Cord PPI network database75 indicates that, as a tendency, they tend to establish more PPI than the average human proteome (Table S6).
From an evolutionary point of view, the fact that the detected amyloid cores accept been not purged out past natural choice back up their functional role, since there is a strong selective pressure to reduce the amyloidogenic load of poly peptide sequences, specially when they are located in disordered regions and thus exposed to solvent76. The presence of this kind of regions is inherently risky, since mutations that would increment their amyloid potency, making them more similar to classical pathogenic amyloid stretches, can promote irreversible protein aggregation, generating a deleterious phenotype resulting from deregulation of the human or heterotypic PPIs in which they are involved. This is the case of hnRNPA1 and hnRNPA2, two human prion-like RNA-binding proteins in which point mutations mapping exactly in the pWALTZ predicted soft amyloid core increment the aggregation propensity of their PrLDs, causing the loss of their regulatory action and leading to the onset of multisystem proteinopathy and ALS14,27. Information technology is worth to explore whether the link to disease of the nucleic acid-binding human proteins in our subproteome and specifically of the seven polypeptides we studied here owes to a related machinery. This would imply that a common process of mutation driven miss-assembly of cryptic amyloid cores in PrLDs might account for very different pathological phenotypes, from cancer to neurodegenerative disorders, depending on the affected protein and the pathways information technology regulates.
Methods
Computational identification of PrLDs in human proteins
The human reference proteome dataset was downloaded from Uniprot77 and scanned for PrLDs using PAPA12 with the default parameters. From the initial 70940 proteins in the proteome, 663 prion-like candidates were identified. Their putative PrLDs were further evaluated with pWALTZ14 with a threshold of 65 in society to identify those domains containing a soft amyloid cadre, which resulted in 535 final positive predictions. The protein sequences selected for experimental characterization were also analyzed with PLAAC16 to define the boundaries of their PrLDs.
Peptide training
We obtained the sequence of the 21 amino acid core region as predicted past pWALTZ14 for the seven poly peptide candidates selected for experimental characterization. The correspondent peptides were purchased from CASLO ApS (Scion Denmark Technical University). The lyophilized peptides were solubilized at a final concentration of 5 mM in dimethyl sulfoxide (DMSO) or in hexafluoro-two-propanol for CD analysis, in order to avoid the large increase in voltage caused by balance DMSO. Correct before each experiment, the stock solutions were diluted to 100 μM in five mM sodium phosphate buffer pH 7,iv. For assemblage assays the samples were incubated for ii days at 37 °C with continuous agitation at 150 rpm in the presence of Teflon beads.
Synchronous lite scattering
Synchronous light handful was monitored using a JASCO Spectrofluorometer FP-8200. The conditions of the spectra acquisition were: excitation wavelength of 360 nm, emission range from 350 to 370 nm, slit widths of 5 nm, 0.5 nm interval and 1000 nm/min browse rate. The peptides were sonicated for ten min in an ultrasonic bath (Fisher Scientific FB15052) before measurement. 100 μl of peptide solution was analyzed.
Bis-ANS (4,4-Dianilino-ane,1-binaphthyl-5,five-disulfonate) binding
The fluorescent spectrum of bis-ANS was analyzed using a JASCO Spectrofluorometer FP-8200. The conditions of the spectra acquisition were: excitation wavelength of 365 nm, emission range from 440 to 640 nm, slit widths of five nm, 0,5 nm interval and yard nm/min scan charge per unit. The peptides were sonicated for 10 min in an ultrasonic bathroom (Fisher Scientific FB15052) before dye addition. 10 µl of peptide solution was added to 100 µl of x µM bis-ANS in HtwoO. A 10 µM bis-ANS solution without peptide was used every bit a control.
Circular dichroism (CD) spectroscopy
CD experiments were performed using a JASCO J-715 spectropolarimeter. Measurements of the far-UV CD spectra (260–190 nm) were fabricated by the addition of 200 µl of the sample to a cuvette of 0.one cm path-length. Spectra were recorded at room temperature, 1 nm band width and 100 nm/min scan rate. The resulting spectrum was the boilerplate of x scans. The contribution of the buffer was subtracted.
Fourier transform infrared (FT-IR) spectroscopy
FTIR experiments were performed using a Bruker Tensor 27 FT-IR spectrometer (Bruker Optics Inc) with a Golden Gate MKII ATR accompaniment. Each spectrum consists of xvi contained scans, measured at a spectral resolution of 4 cm−one within the 1800–1500 cm−1 range. All spectral information were acquired and normalized using the OPUS MIR Tensor 27 software. Data was afterwards deconvoluted using the Top Fit iv.12 program. The buffer without peptide was used as a command and subtracted from the absorbance signal earlier deconvolution.
Manual electron microscopy (TEM)
The morphology of the aggregated peptides was evaluated by negative staining and using a JEOL JEM-1400Plus Transmission Electron Microscope. 5 µl of peptide solution was placed on carbon-coated copper grids and incubated for 5 min. The grids were then washed and stained with 5 µl of 2% w/five uranyl acetate for 5 min. Then, grids were washed once again before assay.
Congo reddish (CR) binding
CR binding to aggregated peptides was analyzed using a Specord® 200 Plus spectrophotometer (Analyticjena). The absorbance spectra were recorded from 400 to 650 nm. Spectra were acquired at 50 nm/sec browse rate. Peptides were sonicated for 10 min in an ultrasonic bath (Fisher Scientific FB15052) before dye addition. 10 μl of the sonicated aggregated peptide was added to 100 µl of 5 µM CR in 5 mM sodium phosphate buffer pH vii,4, and was incubated at room temperature for 5 min earlier the measurement. The same buffer with 5 µM CR and without peptide was employed equally a command.
Thioflavin-T (Th-T) binding
The fluorescence spectra of Thursday-T were recorded using a JASCO Spectrofluorometer FP-8200. The weather of the spectra acquisition were: excitation wavelength of 440 nm, emission range from 460 to 600 nm, slit widths of 5 nm, 0,v nm interval and 1000 nm/min scan rate. Peptides were sonicated for x min in an ultrasonic bathroom (Fisher Scientific FB15052) earlier dye improver. 5 µl of the sonicated aggregated peptide was added to 100 µl of 25 µM ThT in v mM sodium phosphate buffer pH 7,four. The same buffer with 25 µM ThT and without peptide was employed every bit a control.
Thioflavin-S (Th-S) staining
First, 150 µl of aggregated peptides were incubated for 1 h in the presence of 125 µM of ThS in five mM sodium phosphate buffer pH seven,iv. Then, the samples were washed two times with the same buffer. Finally, the precipitated fraction was resuspended in a final volume of x µl and placed on a microscope slide and sealed. Images of the peptide aggregates bound to Th-S were obtained at 40-fold magnification in a Leica fluorescence microscope (Leica DMRB).
Aggregation kinetics and seeding assays
Reactions were carried out at of 100 μM final soluble peptide concentration in a solution containing 25 μM of Th-T at 37 °C in the absence or presence of 2% seeds nether quiescent conditions. The assemblage kinetics were followed monitoring the changes in Th-T fluorescence intensity at 488 nm over the time using a JASCO Spectrofluorometer FP-8200. Before each measure out, the sample was mixed by pipetting upwards and down. The conditions of the spectra conquering were: excitation wavelength of 440 nm, emission range from 460 to 600 nm, slit widths of v nm, 0,5 nm interval and 1000 nm/min browse rate. The seeds were prepared by sonicating the preformed aggregates of the corresponding peptide for 10 min in an ultrasonic bath (Fisher Scientific FB15052) before addition.
Cell viability assay
Human SH-SY5Y cells were seeded into 96-well tissue civilization plate with a density of 4,000 cells/well (100 µL/well) in F-12 medium supplemented with 10% FBS, and maintained at 37 °C and five% CO2 atmosphere. Cell cultures were incubated in the presence of different concentrations of peptides for 72 hours. To command cells, the same volume of PBS1x was added. Following incubation, cells were stained by adding 20 µL PrestoBlue® Jail cell Viability Reagent (Invitrogen) directly to the sample wells. After 30 min of incubation, cell viability was adamant by measuring fluorescence exciting at 531 nm and collecting emission at 615 nm in a Victor fluorescent plate reader (Perkin Elmer).
References
-
Chiti, F. & Dobson, C. One thousand. Poly peptide misfolding, functional amyloid, and hauman disease. Almanac Review of Biochemistry 75, 333–366 (2006).
-
Ventura, S. et al. Short amino acrid stretches can mediate amyloid germination in globular proteins: the Src homology 3 (SH3) case. Proc. Natl. Acad. Sci. United states 101, 7258–7263 (2004).
-
Rousseau, F., Serrano, 50. & Schymkowitz, J. Due west. How evolutionary pressure against poly peptide aggregation shaped chaperone specificity. J Mol Biol 355, 1037–1047 (2006).
-
Aguzzi, A. & Calella, A. Chiliad. Prions: protein aggregation and infectious diseases. Physiological reviews 89, 1105–1152 (2009).
-
Sikorska, B. & Liberski, P. P. Human prion diseases: from Kuru to variant Creutzfeldt-Jakob illness. Sub-cellular biochemistry 65, 457–496 (2012).
-
Si, M. P. What Are They Good For? Annual Review of Cell and Developmental Biological science 31, annurev-cellbio-100913-013409 (2015).
-
Molliex, A. et al. Stage separation by low complexity domains promotes stress granule associates and drives pathological fibrillization. Cell 163, 123–133 (2015).
-
Chakrabortee, S. et al. Intrinsically Disordered Proteins Drive Emergence and Inheritance of Biological Traits Article Intrinsically Disordered Proteins Drive Emergence and Inheritance of Biological Traits. Cell 167, 1–13 (2016).
-
Alberti, S., Halfmann, R., King, O., Kapila, A. & Lindquist, S. A Systematic Survey Identifies Prions and Illuminates Sequence Features of Prionogenic Proteins. Cell 137, 146–158 (2009).
-
Michelitsch, M. D. & Weissman, J. South. A census of glutamine/asparagine-rich regions: implications for their conserved function and the prediction of novel prions. Proceedings of the National University of Sciences of the The states of America 97, 11910–11915 (2000).
-
Harrison, P. M. & Gerstein, M. A method to appraise compositional bias in biological sequences and its awarding to prion-like glutamine/asparagine-rich domains in eukaryotic proteomes. Genome biology 4, R40 (2003).
-
Toombs, J. a. et al. De novo design of constructed prion domains. Proceedings of the National Academy of Sciences 109, 6519–6524 (2012).
-
Espinosa Angarica, Five. et al. PrionScan: an online database of predicted prion domains in consummate proteomes. BMC genomics fifteen, 102 (2014).
-
Sabate, R., Rousseau, F., Schymkowitz, J. & Ventura, S. What Makes a Poly peptide Sequence a Prion? PLoS Computational Biology eleven, e1004013 (2015).
-
Zambrano, R. et al. PrionW: a server to identify proteins containing glutamine/asparagine rich prion-like domains and their amyloid cores. Nucleic Acids Enquiry, 1–7, (2015).
-
Lancaster, A. Thou., Nutter-Upham, A., Lindquist, S. & Male monarch, O. D. PLAAC: A spider web and command-line application to identify proteins with prion-like amino acid composition. Bioinformatics 30, 2501–2502 (2014).
-
Batlle, C., Iglesias, Five., Navarro, South. & Ventura, S. Prion-similar proteins and their computational identification in proteomes. Expert Rev Proteomics 14, 335–350 (2017).
-
King, O. D., Gitler, A. D. & Shorter, J. The tip of the iceberg: RNA-binding proteins with prion-like domains in neurodegenerative disease. Encephalon Research 1462, 61–80 (2012).
-
An, 50. & Harrison, P. One thousand. The evolutionary scope and neurological disease linkage of yeast-prion-like proteins in humans. Biology directly eleven, 32 (2016).
-
Malinovska, L., Palm, S., Gibson, K., Verbavatz, J.-K. & Alberti, S. Dictyostelium discoideum has a highly Q/North-rich proteome and shows an unusual resilience to protein aggregation. Proceedings of the National Academy of Sciences 112, 201504459 (2015).
-
Singh, G. P. et al. Hyper-expansion of asparagines correlates with an abundance of proteins with prion-like domains in Plasmodium falciparum. Molecular and Biochemical Parasitology 137, 307–319 (2004).
-
Espinosa Angarica, 5., Ventura, S. & Sancho, J. Discovering putative prion sequences in complete proteomes using probabilistic representations of Q/Northward-rich domains. BMC genomics 14, i–17 (2013).
-
Chakrabortee, Due south. et al. Luminidependens (LD) is an Arabidopsis protein with prion behavior. Proceedings of the National University of Sciences of the Us of America 113, 201604478 (2016).
-
Kato, M. et al. Jail cell-gratis formation of RNA granules: Low complexity sequence domains grade dynamic fibers within hydrogels. Cell 149, 753–767 (2012).
-
Decker, C. J. & Parker, R. P-bodies and stress granules: possible roles in the control of translation and mRNA degradation. Cold Leap Harbor perspectives in biological science four, a012286 (2012).
-
Patel, A. et al. A Liquid-to-Solid Phase Transition of the ALS Poly peptide FUS Accelerated by Disease Mutation. Cell 162, 1066–1077 (2015).
-
Kim, H. J. et al. Mutations in prion-like domains in hnRNPA2B1 and hnRNPA1 cause multisystem proteinopathy and ALS. Nature 495, 467–473 (2013).
-
Fernandez, M. R., Batlle, C., Gil-Garcia, M. & Ventura, Southward. Amyloid cores in prion domains: Key regulators for prion conformational conversion. Prion eleven, 31–39 (2017).
-
Sabate, R., Rousseau, F., Schymkowitz, J., Batlle, C. & Ventura, S. Amyloids or prions? That is the question. Prion 9, 200–206 (2015).
-
Sant'Anna, R. et al. Characterization of Amyloid Cores in Prion Domains. Scientific Reports half-dozen, 34274 (2016).
-
Pallarès, I., Iglesias, V. & Ventura, South. The rho termination factor of Clostridium botulinum contains a prion-like domain with a highly amyloidogenic cadre. Frontiers in Microbiology 6, one–12 (2016).
-
Yuan, A. H. & Hochschild, A. A bacterial global regulator forms a prion. Science 355, 198–201 (2017).
-
Li, X., Rayman, J. B., Kandel, East. R. & Derkatch, I. Fifty. Functional Role of Tia1/Pub1 and Sup35 Prion Domains: Directing Poly peptide Synthesis Mechanism to the Tubulin Cytoskeleton. Molecular Cell 55, 305–318 (2014).
-
Fuller-Pace, F. Five. The Expressionless box proteins DDX5 (p68) and DDX17 (p72): Multi-tasking transcriptional regulators. Biochimica et Biophysica Acta - Gene Regulatory Mechanisms 1829, 756–763 (2013).
-
Janknecht, R. Multi-talented expressionless-box proteins and potential tumor promoters: P68 RNA helicase (DDx5) and its paralog, p72 RNA helicase (DDx17). American Journal of Translational Research ii, 223–234 (2010).
-
Mazurek, A. et al. DDX5 regulates DNA replication and is required for jail cell proliferation in a subset of breast cancer cells. Cancer discovery ii, 812–825 (2012).
-
Fuller-Pace, F. V. The Dead box proteins DDX5 (p68) and DDX17 (p72): multi-tasking transcriptional regulators. Biochim Biophys Acta 1829, 756–763 (2013).
-
Xu, J. et al. Eya1 interacts with Six2 and Myc to regulate expansion of the nephron progenitor pool during nephrogenesis. Developmental Cell 31, 434–447 (2014).
-
Wu, Thousand. et al. EYA1 phosphatase function is essential to drive breast cancer cell proliferation through cyclin D1. Cancer Res 73, 4488–4499 (2013).
-
Buller, C., Xu, X., Marquis, V., Schwanke, R. & Xu, P. Ten. Molecular furnishings of Eya1 domain mutations causing organ defects in BOR syndrome. Man molecular genetics x, 2775–2781 (2001).
-
Xu, P. 10., Cheng, J., Epstein, J. A. & Maas, R. L. Mouse Eya genes are expressed during limb tendon development and encode a transcriptional activation function. Proc Natl Acad Sci United states of america 94, 11974–11979 (1997).
-
Castella, S., Bernard, R., Corno, M., Fradin, A. & Larcher, J. C. Ilf3 and NF90 functions in RNA biology. Wiley Interdisciplinary Reviews: RNA half dozen, 243–256 (2015).
-
Shiina, N. & Nakayama, G. RNA granule associates and disassembly modulated by nuclear factor associated with double-stranded RNA ii and nuclear factor 45. The Journal of biological chemistry 289, 21163–21180 (2014).
-
Saunders, L. R. et al. Characterization of two evolutionarily conserved, alternatively spliced nuclear phosphoproteins, NFAR-1 and -two, that function in mRNA processing and interact with the double-stranded RNA-dependent protein kinase, PKR. The Periodical of biological chemical science 276, 32300–32312 (2001).
-
Wang, X. et al. Redefining the modular system of the core Mediator complex. Prison cell Enquiry 24, 796–808 (2014).
-
Halfmann, R. et al. Opposing Furnishings of Glutamine and Asparagine Govern Prion Formation past Intrinsically Disordered Proteins. Molecular Prison cell 43, 72–84 (2011).
-
Zhu, 10. et al. Mediator tail subunits tin form amyloid-like aggregates in vivo and bear upon stress response in yeast. Nucleic Acids Inquiry 43, 7306–7314 (2015).
-
Xu, J. & Li, Q. Review of the in vivo functions of the p160 steroid receptor coactivator family. Molecular Endocrinology 17, 1681–1692 (2003).
-
Silva, Grand. P. et al. NCOA2 is a candidate target factor of 8q gain associated with clinically aggressive prostate cancer. Genes Chromosomes and Cancer 55, 365–374 (2016).
-
Suresh, S. et al. SRC-ii-mediated coactivation of anti-tumorigenic target genes suppresses MYC-induced liver cancer. PLoS genetics thirteen, e1006650 (2017).
-
Armada, T. et al. SRC-2 orchestrates polygenic inputs for fine-tuning glucose homeostasis. Proceedings of the National Academy of Sciences of the United states of america of America 112, E6068–6077 (2015).
-
Sparmann, A. & van Lohuizen, M. Polycomb silencers control cell fate, development and cancer. Nature reviews. Cancer vi, 846–856 (2006).
-
Awad, Southward. et al. Mutation in PHC1 implicates chromatin remodeling in main microcephaly pathogenesis. Man Molecular Genetics 22, 2200–2213 (2013).
-
Conchillo-Sole, O. et al. AGGRESCAN: a server for the prediction and evaluation of "hot spots" of assemblage in polypeptides. BMC bioinformatics 8, 65 (2007).
-
Fernandez-Escamilla, A.-Thousand., Rousseau, F., Schymkowitz, J. & Serrano, L. Prediction of sequence-dependent and mutational effects on the aggregation of peptides and proteins. Nature biotechnology 22, 1302–1306 (2004).
-
Tartaglia, G. G. & Vendruscolo, M. The Zyggregator method for predicting protein aggregation propensities. Chemic Society reviews 37, 1395–1401 (2008).
-
Prilusky, J. et al. FoldIndex©: A simple tool to predict whether a given protein sequence is intrinsically unfolded. Bioinformatics 21, 3435–3438 (2005).
-
Dosztanyi, Z., Csizmok, V., Tompa, P. & Simon, I. IUPred: web server for the prediction of intrinsically unstructured regions of proteins based on estimated energy content. Bioinformatics 21, 3433–3434 (2005).
-
Xue, B., Dunbrack, R. L., Williams, R. W., Dunker, A. K. & Uversky, V. N. PONDR-FIT: a meta-predictor of intrinsically disordered amino acids. Biochimica et biophysica acta 1804, 996–1010 (2010).
-
Yang, Z. R., Thomson, R., McNeil, P. & Esnouf, R. M. RONN: the bio-basis function neural network technique applied to the detection of natively disordered regions in proteins. Bioinformatics 21, 3369–3376 (2005).
-
de Groot, Northward. S., Parella, T., Aviles, F. X., Vendrell, J. & Ventura, Due south. Ile-phe dipeptide self-assembly: clues to amyloid germination. Biophys. J. 92, 1732–1741 (2007).
-
Klunk, Due west. E., Pettegrew, J. W. & Abraham, D. J. Quantitative evaluation of congo cherry-red binding to amyloid-similar proteins with a beta-pleated sheet conformation. J. Histochem. Cytochem. 37, 1273–1281 (1989).
-
Sabate, R., Rodriguez-Santiago, L., Sodupe, 1000., Saupe, S. J. & Ventura, S. Thioflavin-T excimer germination upon interaction with amyloid fibers. Chem. Commun. (Camb.) 49, 5745–5747 (2013).
-
Wickner, R. B. et al. Yeast prions act as genes composed of cocky-propagating protein amyloids. Adv. Poly peptide Chem. 57, 313–334 (2001).
-
Jarrett, J. T. & Lansbury, P. T. Jr. Seeding "one-dimensional crystallization" of amyloid: a pathogenic mechanism in Alzheimer's disease and scrapie? Prison cell 73, 1055–1058 (1993).
-
Pastor, M. T., Esteras-Chopo, A. & Serrano, Fifty. Hacking the lawmaking of amyloid formation: the amyloid stretch hypothesis. Prion i, 9–14 (2007).
-
Sabate, R., Espargaro, A., Grana-Montes, R., Reverter, D. & Ventura, Southward. Native structure protects SUMO proteins from aggregation into amyloid fibrils. Biomacromolecules 13, 1916–1926 (2012).
-
Liebman, S. W. & Chernoff, Y. O. in Genetics Vol. 191, 1041–1072 (Genetics, 2012).
-
Kato, Yard. et al. Cell-free formation of RNA granules: depression complication sequence domains grade dynamic fibers within hydrogels. Cell 149, 753–767 (2012).
-
Kato, M. & Mcknight, Southward. L. Cross- b Polymerization of Low Complication Sequence Domains. ane–11, (2016).
-
Gilks, Due north. et al. Stress granule assembly is mediated past prion-like assemblage of TIA-one. Molecular biology of the jail cell xv, 5383–5398 (2004).
-
Kanai, Y., Dohmae, Due north. & Hirokawa, N. Kinesin transports RNA: isolation and label of an RNA-transporting granule. Neuron 43, 513–525 (2004).
-
Elvira, Grand. et al. Label of an RNA granule from developing encephalon. Molecular & cellular proteomics: MCP 5, 635–651 (2006).
-
Kwon, I. et al. Phosphorylation-regulated binding of RNA polymerase II to fibrous polymers of low-complexity domains. Jail cell 155, 1049–1060 (2013).
-
Szklarczyk, D. et al. String v10: protein-protein interaction networks, integrated over the tree of life. Nucleic Acids Res 43, D447–452 (2015).
-
Monsellier, Due east. & Chiti, F. Prevention of amyloid-like aggregation as a driving force of protein development. EMBO Rep. 8, 737–742 (2007).
-
UniProt Consortium, T. U. UniProt: a hub for poly peptide information. Nucleic acids enquiry 43, D204-212 (2015).
-
Rappaport, N. et al. MalaCards: an amalgamated man disease compendium with diverse clinical and genetic annotation and structured search. Nucleic Acids Res 45, D877–D887 (2017).
Acknowledgements
This work was funded supported by the Spanish Ministry of Economy and Competitiveness [BIO2016-783-78310-R to Due south.V] and by ICREA [ICREA-Academia 2015 to S.V.]
Author information
Affiliations
Contributions
Conceived and supervised: Due south.V. Performed the experiments: C.B., 5.I. and S.N. Design, analysis and estimation of experiments: C.B., N.S.G. and S.V. Wrote the paper: C.B. and S.Five.
Respective writer
Ethics declarations
Competing Interests
The authors declare that they take no competing interests.
Additional information
Publisher'due south note: Springer Nature remains neutral with regard to jurisdictional claims in published maps and institutional affiliations.
Electronic supplementary textile
Rights and permissions
Open Access This article is licensed nether a Creative Commons Attribution four.0 International License, which permits use, sharing, adaptation, distribution and reproduction in any medium or format, as long as you give appropriate credit to the original author(s) and the source, provide a link to the Artistic Commons license, and indicate if changes were made. The images or other third party fabric in this article are included in the article'south Creative Commons license, unless indicated otherwise in a credit line to the fabric. If material is not included in the article's Creative Eatables license and your intended utilize is not permitted by statutory regulation or exceeds the permitted utilize, you will need to obtain permission directly from the copyright holder. To view a copy of this license, visit http://creativecommons.org/licenses/by/4.0/.
Reprints and Permissions
About this article
Cite this article
Batlle, C., de Groot, N.S., Iglesias, V. et al. Characterization of Soft Amyloid Cores in Human Prion-Like Proteins. Sci Rep 7, 12134 (2017). https://doi.org/10.1038/s41598-017-09714-z
-
Received:
-
Accepted:
-
Published:
-
DOI : https://doi.org/ten.1038/s41598-017-09714-z
Further reading
Comments
By submitting a comment y'all agree to bide by our Terms and Customs Guidelines. If you find something abusive or that does non comply with our terms or guidelines please flag information technology as inappropriate.
Source: https://www.nature.com/articles/s41598-017-09714-z
0 Response to "what criteria would you use to determine if a protein was exhibiting prion properties?"
Post a Comment